Today we are going to be talking to Professor Fred Hagen from the Delft University of Technology and learning about his discovery of an extraordinary and unique analytical signature in electron paramagnetic resonance (EPR) spectroscopy that proves the existence of hydrino.
Top takeaways:
An reputable biochemist and expert in EPR spectroscopy, Fred Hagen, has experimentally confirmed the existence of hydrino.
Hydrogen molecules are diamagnetic, but “hydrino” molecules are shown to be paramagnetic. This creates a clear test for the existence of hydrino.
Paramagnetic hydrino gas was trapped in microspherical cages of gallium oxide hydroxide, and resulted in a gas-phase EPR spectra from a solid powder sample. This study was published in 2022.
A follow up experiment found solid-state EPR spectra from numerous samples prepared in a variety of ways, as well as a unique temperature-dependent behavior. This study has been submitted for publication.
The temperature dependence is explained by how hydrino molecules form end-to-end dimers due to their paramagnetism as well as dimers-of-dimers at low temperature due to dipolar interactions.
Due to the pervasive attitude of skepticism toward hydrino research, Hagen’s first paper took 16 months to publish. “As a scientific community we cannot afford to ignore this.”
The following is a transcript of the podcast, which includes excerpts from interviews with Hagen conducted in January of 2023 and January of 2025.
BH: In 1990, Randell Mills predicted the existence of a new kind of hydrogen atom and molecule called “hydrino.” In hydrino atoms, the electron is more tightly bound to the proton, resulting in a physically smaller and chemically inert species. For decades, Mills and his team, as well as collaborating and consulting scientists, have been pioneering a new field of hydrogen chemistry, learning how to make hydrino, and gathering compelling analytical evidence for it. But there's been a lot of skepticism, particularly by theoreticians who claim that hydrino atoms shouldn't exist, according to quantum mechanics.
Although Mills and his team have published their findings widely in scientific journals, high-impact journals generally abstain from publishing on the topic, and there is this lingering aura of doubt. It's not clear that anyone realizes how much evidence has been submitted, and everyone seems to assume that it's been debunked, by someone, at some point. Everyone is waiting for everyone else to pay attention.
I've been following this topic a long time. A popular refrain we hear over and over again is, “Extraordinary claims require extraordinary evidence.” It was a popular notion by Carl Sagan, and often invoked when he was talking about things like UFOs. But this is not a UFO; this is a hydrogen molecule that can be subjected to analysis.
In practice, Sagan's aphorism has meant that anyone can choose to ignore any evidence that they feel is not “extraordinary” enough.
Today, we're going to speak to Professor Fred Hagen, who has conducted an independent analysis of hydrino molecules that has resulted in an extraordinary and unique signal.
So, let's meet Fred Hagen.
FH: Well, my name is Fred Hagen. I am 73 years old. I am an emeritus professor in biochemistry. I was born and raised in Amsterdam. That's also where I studied and where I did my PhD. After that, I spent a few years in the US at the University of Michigan in Ann Arbor. And then I returned to the Netherlands, first to the Wageningen University, where I was an assistant professor in biochemistry, later associate professor, and finally a professor of bioinorganic chemistry.
Then I also became a professor of physical chemistry at the Nijmegen University. And finally, around 2000, I decided that this was all too much to combine and I moved to the University of Technology of Delft.
BH: Hagen is an expert in "Electron Paramagnetic Resonance” (EPR) spectroscopy.
FH: Well, EPR spectroscopy requires systems that have unpaired electrons, which for chemists are mainly two groups. One is radicals, maybe mainly organic radicals. But the other group was more of my interest, and that is transition ions like iron and manganese and copper and stuff like that. So those can be measured with EPR and I measure them because they occur in biomolecules, in particular in enzymes.
BH: In this process, a sample is placed under a magnetic field, and light is shined on the sample over a range of frequencies. If the sample has unpaired electrons, it will align itself to the magnetic field. But if it absorbs the right energy of light, it will flip from one orientation to another, and the absorption of this light is detected.
FH: Although officially retired, I still work every day, not so much in the lab anymore, maybe once a week, maximally. I spent some of my time, maybe 20% or so, to help other people out with their problems in case electron paramagnetic fluorescence or EPR spectroscopy could be of any help.
I have a broad interest in things, anything that has to do with science. And I like to try things out. So anybody who comes to me with a problem that I don't know about, which is interesting, then I'll spend some time trying to see whether I can be of any contribution.
BH: Hagen was retired and liked to intersperse research projects for other scientists with his own. Then one day someone came into Hagen's office with an interesting project.
FH: I think it was 2019. In the autumn of 2019, a Dutch guy called Peter van Norden came into my office.
BH: Van Norden was a retired radiologist who had followed Mills for 20 years. During that time, he had connected Mills to other scientists in the Netherlands, including Gerrit Kroesen at the Technical University Eindhoven. And Kroesen had replicated a number of Mills' plasma experiments back in the 2000s.
FH: And he asked me to do a few trial experiments on the samples that were produced by Mills, samples containing hydrino, which Mills predicted to be paramagnetic. And paramagnetic is the property that we need in order to be able to do this EPR spectroscopy.
And that is very unusual because normally molecular hydrogen, the H2 molecule, has two electrons which are paired. So each electron is magnetic, but once they are paired, the magnetism is zeroed. So a hydrogen molecule that is paramagnetic, that would be very, very unusual.
BH: Mills has an entirely new theory for atoms and molecules that uses classical electrodynamics. Electrons in the atom are spherical shells. Electrons in a molecular bond are ellipsoidal shells. Because electrons obey classical electrodynamics, [electrons in the hydrogen atom] can undergo a “resonant energy transfer” reaction that allows them to shrink to a “hydrino” state.
According to Mills' theory, hydrino atoms are an integer fraction of the size of a normal hydrogen atom at H(1/2), H(1/3), H(1/4)… etc. When they bond to form a hydrino molecule, they have a shorter interatomic distance, (again) at a fractional size of the hydrogen molecule.
When two hydrogen atoms bond, the electrons pair up in the new molecule. But when two hydrino atoms bond, they each bring with them an extra ‘captured’ photon. Half of each electron combines with each photon in the same direction, but the other half of each electron combines with each photon in opposite directions.
This is really weird.
FH: One half up, one half up, and one half up, one half down. But one half electron doesn't exist because you cannot split an electron. And therefore we end up with one whole electron paramagnetic and one whole electron diamagnetic, which is the whole crux of the story.
I must admit that at first I was rather skeptical about this whole proposal because I'd never heard of a hydrogen molecule being paramagnetic. So as soon as I really got interested, then I contacted him and I said, I want to do this completely independently. I want to be an independent scientist, which is the best way I think I can help you. So I will not accept any money from you. And that also implies that I don't accept any directives; I just do what I wanted to do and just take it or leave it.
“I must admit that at first I was rather skeptical about this whole proposal because I'd never heard of a hydrogen molecule being paramagnetic.”
And the first few samples that I measured were not very promising because they contained all kinds of lines. But many of them I could identify as metal contaminations. But I continued for a while, and after a few weeks, I got samples that were much more pure. And one day I was looking at a sample and it was really clean. It had a very flat baseline over the whole range that the spectrometer could cover except for one very sharp line. And so I got into that a little bit more in into the details and it turned out that that very sharp line actually consisted of many, many lines with very, very sharp line widths. A line width that was so sharp that I had never seen [anything like] it before.
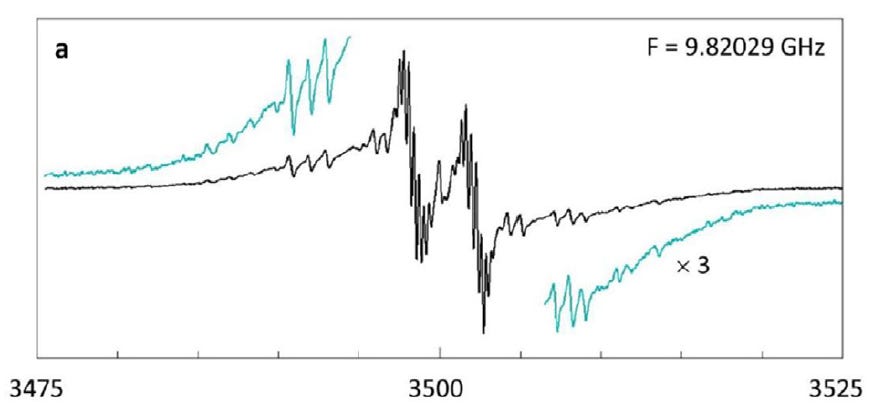
It was a little bit difficult to measure that spectrum because it was rather weak. So it took unusually long. Normally when I take a spectrum, it takes a few minutes. This time to completely resolve the whole spectrum required several hours of accumulating data.
BH: But hours he had. This was 2020, and it was the beginning of the pandemic shutdown. The laboratory building, designed to accommodate 2,000 people, was empty. Aside from a security guard and a cleaning lady, he was alone in the lab. Instead of running a sample for minutes, he could set it up to run for days.
FH: Actually, for good spectra, it took a few days to collect one spectra.
BH: 40 hours on average. He did this for months.
FH: Well, first of all, the line width of each individual line was very small. It was like 0.17 Gauss. And that is something in my own work I'd never seen before. So I went through the literature to try to find what systems could have small, very small line width. And there were a few, but they were not really an explanation of what I was looking at, except for one possibility, and that was gas phase molecules.
Now, as a biochemist interested in proteins, I never studied gas phase molecules.
And actually, it turned out to be a rather small niche of the whole literature of EPR spectroscopy. Perhaps in total, 20 molecules were ever studied in the gas phase with EPR. But in those systems, yes, you could see lines that were pretty sharp. So what they suggested was that what I was looking at was possibly a gas phase molecule.
Now, these samples are solids. The material that we used to catch this hydrogen actually formed small spheres in the solid state in which these hydrogen molecules were included. And apparently, according to the EPR, this suggested that they were in the gas phase.
BH: Hagen was seeing a sharp line from a gas sample in a solid powder. To prepare the sample, Mills' team had used one of their closed reaction vessels containing liquid gallium.
FH: This paper was produced with a very particular electrode in a plasma reactor, and the electrode was gallium.
BH: Since gallium would interfere with EPR, they were able to separate out a high-purity crystalline gallium oxide hydroxide for analysis. It turned out that this gallium oxide hydroxide powder had formed fibers consisting of chains of micro-spherical particles about 100 nanometers in width, made of polymeric crystals in a hexagonal structure. These spheres had trapped small quantities of the hydrino gas. It was a gas sample trapped in a powder. Since hydrino is much smaller than conventional hydrogen gas, it's very difficult to simply store it in a bottle. The best way to analyze it is to trap it on a molecular level.
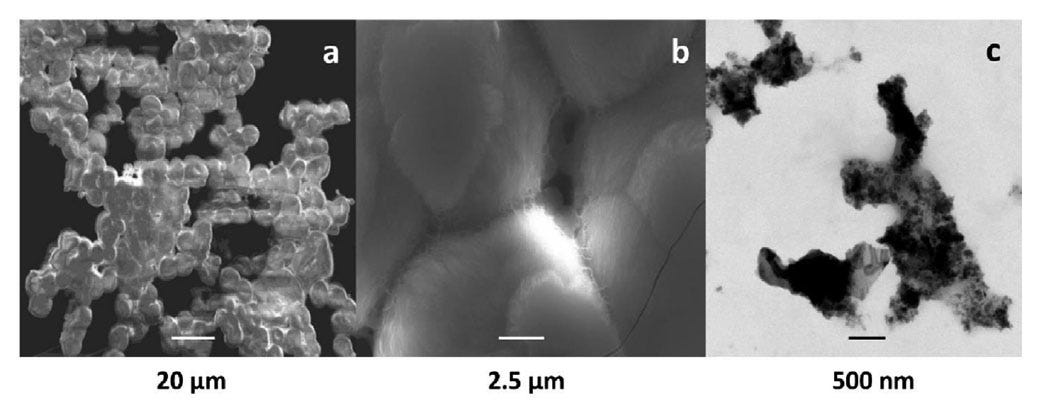
FH: And apparently that was the material. (Well, not apparently, we could prove that chemically. That was the material that included the hydrino and that gave this particular structure.
Well, at the end of [these] months, I was really completely converted to a believer in hydrino because these data were so incredibly unusual. And at the same time, they were predicted to be paramagnetic and they were paramagnetic. Also [they were] predicted to have a certain structure, which I found. And then all the details were later also explained.
“I was really completely converted to a believer in hydrino because these data were so incredibly unusual.”
BH: When I first interviewed Hagen in 2023, he had completed his first experimental paper, and in addition to a complex multi-line EPR spectra, Mills's team also performed a variety of other spectroscopic studies on the material. When they performed gas chromatography, they found that the hydrino gas has a much faster migration rate than normal hydrogen gas. This is because it has 1/64th of the “ballistic” cross section. It's a lot smaller.
They also performed energy dissipative x-ray spectroscopy, Rutherford backscattering spectroscopy, time of flight secondary ion mass spectroscopy, and selected area electron diffraction, as well as x-ray diffraction.
The EPR spectrum that Hagen found closely matched Mills' predictions. Mills [and Hagen were] able to produce a theoretical simulation of the structure that closely matched what was found. The difference between the theoretical and experimental peak positions was really small, within experimental uncertainty.
The team produced identical signatures in 11 samples and also ran another EPR spectrum in the United States. When Hagen investigated the alternative explanation using standard quantum mechanics:
FH: It's possible to reproduce a spectrum, assuming a very complicated structure, but it's an extremely unlikely interpretation.
BH: He found that this signal would have to be formed by radicals with considerable complexity, but they would also have to be produced from only hydrogen, oxygen, water, and gallium. And these options were eliminated by Mills' other experimental work. They also performed an analysis with deuterium instead of hydrogen, and the results were as expected.
FH: So I ended up with a bunch of data with a rather detailed explanation that fitted those data. And so I said, “Well, I think I found something interesting. I should tell the world about this.” So together with Randy, I wrote a paper about this.
BH: Hagen's paper was a monumental experimental confirmation of a state of matter that might rewrite our atomic theory. In the conclusion, Hagen said it was “an urgent invitation to academia at large to repeat and extend the described experiments in lieu of refutation on quantum mechanical theoretical grounds.” It meant that if physicists were critical of the model, they would have to go into the laboratory and see for themselves.
FH: Nobody else in the world believes this, except for a few people like you. So it turned out to be incredibly difficult to get this manuscript published. I started with a few very high impact journals, and they typically immediately returned the manuscript within a few days and said, something like: “No, this is not really the type of thing that we publish” without any explanation. And so I went down a little bit to another journal with a little bit lower impact factor and so on and so forth.
And it took me altogether approximately 16 months to get it published. But 16 months was, to me, a world record in my career. But anyway, in the end, we were successful.
BH: Two years after their first round of experiments, Hagen and Mills have completed another round of EPR studies [not yet published]. They found similar spectra from samples prepared a wide variety of ways, but there was an important difference.
FH: Now, there are many different ways in which you can produce hydrinos, molecular hydrinos. And Randy has, of course, a multiple of them, but none of them actually reproduced this very specific signal, except in [the gallium] reactors.
The other samples were not produced as gallium as an electrode, but other material as an electrode or by other methods, very different methods than plasma reactors. But they all turned out to have EPR signals, but they were very different in the sense that they were two or three orders of magnitude broader.
At least it tells me that it's definitely not gas molecules that I'm looking at this time, but genuine solid state molecules. The signals looked familiar to me, because I worked for some, I think 10-15 years or so on a biomolecule called ferritin, which is the molecule in our body that stores iron.
So the first thing I did was actually ask Randy to do a number of control experiments to make absolutely sure that there is no iron involved somewhere from the walls of the reactor or whatever. And one of the ways he produced these samples was with a ball mill machine.
So in which he put certain material that should produce the catalyst required for hydrino production and also the hydrogen required for the hydrino reaction, and then mill them for a long time with high intensity, and then that would produce indeed material that contained hydrino, according to a number of control experiments.
But those ball mill machines are usually made of stainless steel. So my first worry was, well, perhaps it's iron from the stainless steel that comes into the sample and we're just looking at the trivial contaminant. So he went to some trouble in purchasing a tungsten ball mill from China, tungsten container and tungsten balls. So everything was tungsten and there was no iron. And that material gave exactly the same EPR spectrum. So for me, that was a proof that at least iron is not involved as a contaminant.
Okay, so we had three different types of ball mill machines and we also had his plasma reactors and they all produce samples that with some variation all approximately gave similar EPR spectra: a very broad line at a specific field position.
It's quite reproducible. It's not just one line. It's more complicated than that because there are shoulders on both sides and there are extra lines at low magnetic field. And that pattern with variations is always reproduced independent of how you make the samples.
BH: Hagen also studied the temperature dependence of the EPR signal, which led to a new fascinating discovery about the nature of hydrino molecules:
FH: That was a crucial experiment. So normally in EPR, you start with room temperature and then you go to lower temperature, cooling with liquid nitrogen and liquid [helium]. because many systems have broad lines at room temperature and they become sharper at lower temperature because the relaxation of the signal from the excited state to the ground state becomes slower, so you get sharp lines. So that's the standard approach.
I did see the signal vary a little bit with temperature, but not change very much in appearance. But it did change very much in intensity, again, in a way that I had never seen before.
So if you take a normal simple EPR sample like a radical, an organic radical, which has one impaired electron, that has a spin of one half, which means that it can have two different energy states in a magnetic field. It can be parallel or it can be anti-parallel to the magnet of the spectrometer. So there are two levels. And the intensity, according to a well-known simple law called the Curie law, tells you that the intensity of the signal should be inversely proportional to the temperature. So at low temperature it has a high intensity, and the higher the temperature the lower the intensity becomes. And the way we plot this usually in EPR literature is to take the amplitude of the sample, multiply it by the temperature, and then it should be a constant. So if you take that product and plot it versus temperature, you get a straight line parallel to the x-axis. Very simple.
In more complicated systems, you usually see that it deviates from linearity. It becomes less intense at higher temperature. That is a sign that you have a high spin system, a system with more than one impaired electron, like metal, transition metals. And ever so often you see that it actually increases, but then decreases again. And that is a sign that you're looking at an excited state.
Now, what I saw was none of those. What I saw was it was very weak at low temperature, starting from 8 Kelvin all the way up to about 100 Kelvin. And then it suddenly jumped up and then it just increased until room temperature, which was the highest temperature that I measured.
I found something, again, that I'd never seen before. Now I've done EPR spectroscopy now for about 50 years or so, so it's a good reference system that I have in my head, but this was completely new.
“I found something, again, that I'd never seen before. Now I've done EPR spectroscopy now for about 50 years or so, so it's a good reference system that I have in my head, but this was completely new.”
Well, the theory that we have right now is that molecular hydrino is a molecule of two hydrino atoms. It's linear, and it has a tendency to form dimers with another molecule [of] hydrino in a linear shape. So along the long axis, it forms a dimer, which is still paramagnetic.
But it also is subject to dipolar interaction, which is a general interaction between magnetic species. Well, it's typical for dipolar interaction that if you have two molecules with a magnetic moment, they force each other into a configuration where the magnetic moments are opposite, so they cancel each other. And so the theory now is that when you have such a dimer, it also couples by dipolar interaction to a second dimer, and then the magnetism is canceled.
So you form a dimer of dimer at low temperature, and then the magnetism is cancelled, and that is why I hardly see any intensity at low temperature. If you increase the temperature, you add energy to the system, and at some point, this energy is enough to break this coupling. And then the single dimers come out, which are paramagnetic.
And the higher the temperature, the more single dimers you get and the more paramagnetism you get. So this all happens in a solid state in which the hydrino molecules are included (in a salt, for example) but they can of course move around a little bit and so they decouple from the dipolar interaction at some temperature and this decoupling increases at the increasing temperature you see the signal increase. That's the theory, for the moment.
I realized that this is looked upon very negatively by many people, but I'm without any prejudices and I just want to start and see what comes out of this. Well, I'm always open to any criticism, if somebody finds another explanation for what I have seen, I'm, of course, very willing to look into that. But I haven't been able myself to come up with an alternative, although I did a bunch of control experiments.
And so for me, for the moment, this is support for the idea that hydrino actually exists.
But I should emphasize that I'm completely independent scientifically from Mills. I have my own opinion, I have to convince myself, which in science should be the most difficult thing to do.
“This is support for the idea that hydrino actually exists.”
BH: Over the decades of research in hydrino chemistry, this is not the first time that the analytical work by Mills and his team have been independently verified by outside parties. Many have merely performed contract experimental work to validate Mills's findings. These groups often do not comment on the wider theoretical implications. Others have closely collaborated with Mills's team on-site, performing some contract work, but also carrying out their own experimental work, independently off-site. Several reputable scientists have spoken out passionately about the impact of their research as well as the pathological, pervasive attitude of skepticism they get from their peers.
In 2009, Peter Jansson concluded a paper with the "sincere hope that other research laboratories across the world will find confidence from our results and begin reproducing these experiments for themselves. That was 16 years ago. If the scientific community has been waiting for extraordinary evidence, it is time to wake up; this is it.
This research has the potential to rewrite quantum theory, bringing the "quantum century" which began in 1925, to an end. This research also has the potential to answer big questions about the nature of the universe.
Hydrino emission lines have been detected in interstellar space. Most of the mass in the galaxy is hydrogen, and the missing mass - "dark matter" that surrounds the galaxy in vast clouds, may also be hydrogen, just in a new form - as hydrino. Most of the mass in the Sun is also hydrogen, and the hydrino reaction may explain a constellation of mysteries there too.
FH: We as a scientific community, as a worldwide scientific community, cannot afford to ignore this. We should do something about it and do something different from what my dear colleagues have done until now, which is only criticizing on the basis of theory. Of course it doesn't fit standard quantum mechanics because it's supposed to be an alternative. It shouldn't fit. What you physicists should do is roll up your sleeves and get into the lab and try to disprove what he says.
Errata:
21:00 FH: “That was a crucial experiment. So normally in EPR, you start with room temperature and then you go to lower temperature, cooling with liquid nitrogen and liquid [helium].
Thoughts and questions? Join the conversation!
For further reading, this topic is covered in the forthcoming book: The End of Fire: how the hydrino is sparking a revolution in physics and clean energy by Brett Holverstott.
Share this post